Hydrogen Production Technology and Processes
Hydrogen has emerged as a promising energy carrier with the potential to play a significant role in the transition towards a more sustainable and low-carbon future. Hydrogen generation technologies are pivotal in the transition towards a sustainable energy future. The technologies for hydrogen generation available in the market today vary widely in terms of their underlying principles, efficiency, cost, and environmental impact.
Hydrogen Production from Natural Gas
Steam Methane Reforming (SMR): Among the established methods,SMR stands out as the most widely used, accounting for approximately 95% of global hydrogen production. SMR involves reacting natural gas with steam at high temperatures to produce hydrogen.
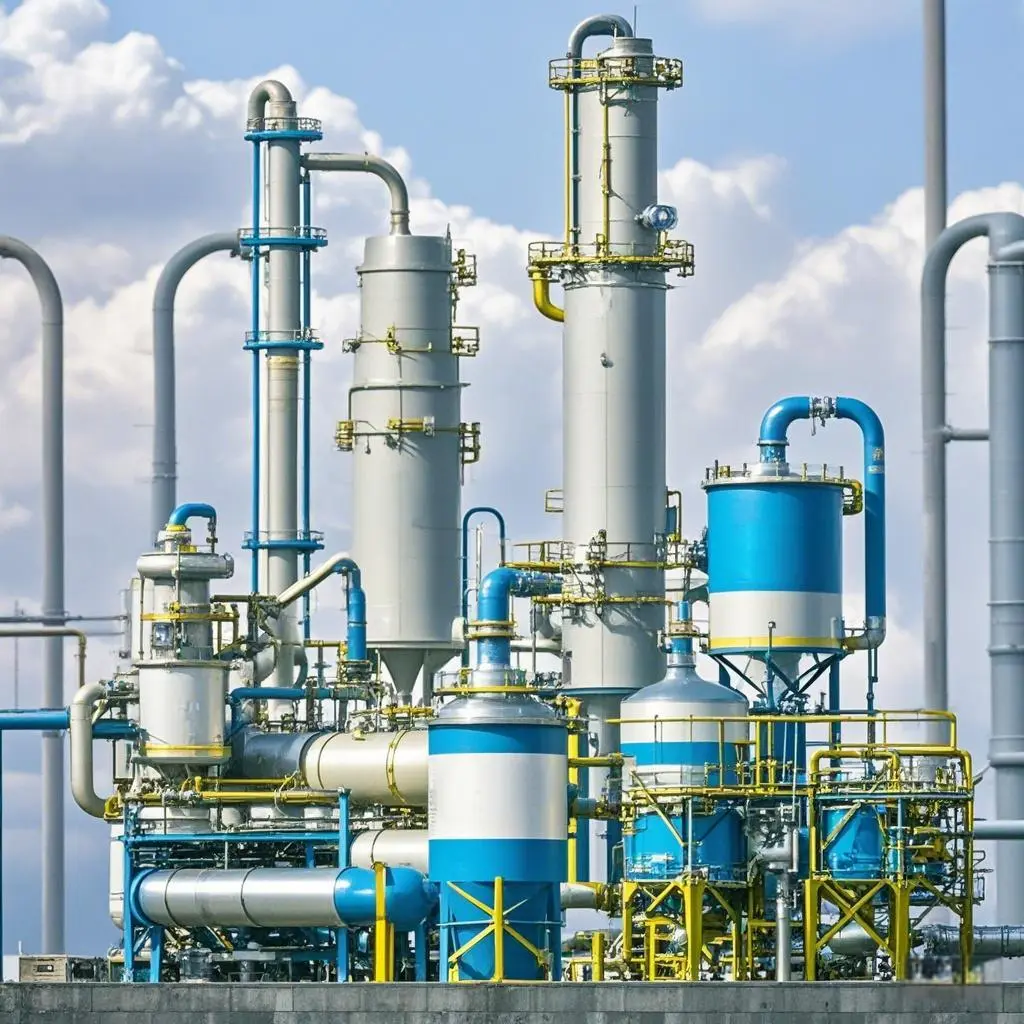
The cost to build an SMR plant ranges from $100 to $200 million, with operational costs varying based on natural gas prices. Maintenance is relatively straightforward due to the technology's maturity, but the environmental impact poses a challenge for scaling up.
Partial Oxidation (POX) is another prominent method, particularly suitable for heavy hydrocarbon feedstocks. POX involves reacting hydrocarbons with oxygen to produce hydrogen, offering the advantage of flexibility in feedstock, including coal and heavy oils. However, it is less efficient than SMR and produces substantial amounts of carbon monoxide, requiring additional processing. The capital cost for a POX plant is similar to SMR, but operational costs can be higher due to the need for pure oxygen. Maintenance is more complex, and the market share is smaller, around 2%, due to its lower efficiency and higher costs.
Autothermal Reforming (ATR) combines SMR and POX processes, using both steam and oxygen to convert hydrocarbons into hydrogen. ATR offers higher efficiency and lower carbon emissions compared to POX, making it a more environmentally friendly option. However, it is more complex and costly to build, with capital costs ranging from $150 to $250 million. Operational costs are also higher due to the need for both steam and oxygen. ATR's market share is modest, around 3%, but it is gaining traction due to its efficiency and lower emissions.
The main challenge for scaling-up these processes is reducing its carbon footprint. Developing carbon capture and storage (CCS) technologies, that can be effectively integrated with production methods extracting hydrogen from feedstocks, is essential but costly and technologically complex. Some refer to hydrogen produced from these fossil fuels feedstocks by using above methods (typically SMR) as “Blue hydrogen” together with CCS.
Hydrogen Production using Electrolysis
Water electrolysis is a clean method of hydrogen production that splits water into hydrogen and oxygen using electricity. It is highly advantageous for its zero-emission potential, especially when powered by electricity from renewable sources such as solar, wind, or hydro. For instance, in regions with abundant wind energy, wind farms can be coupled with electrolysis plants to produce ‘Green hydrogen’.
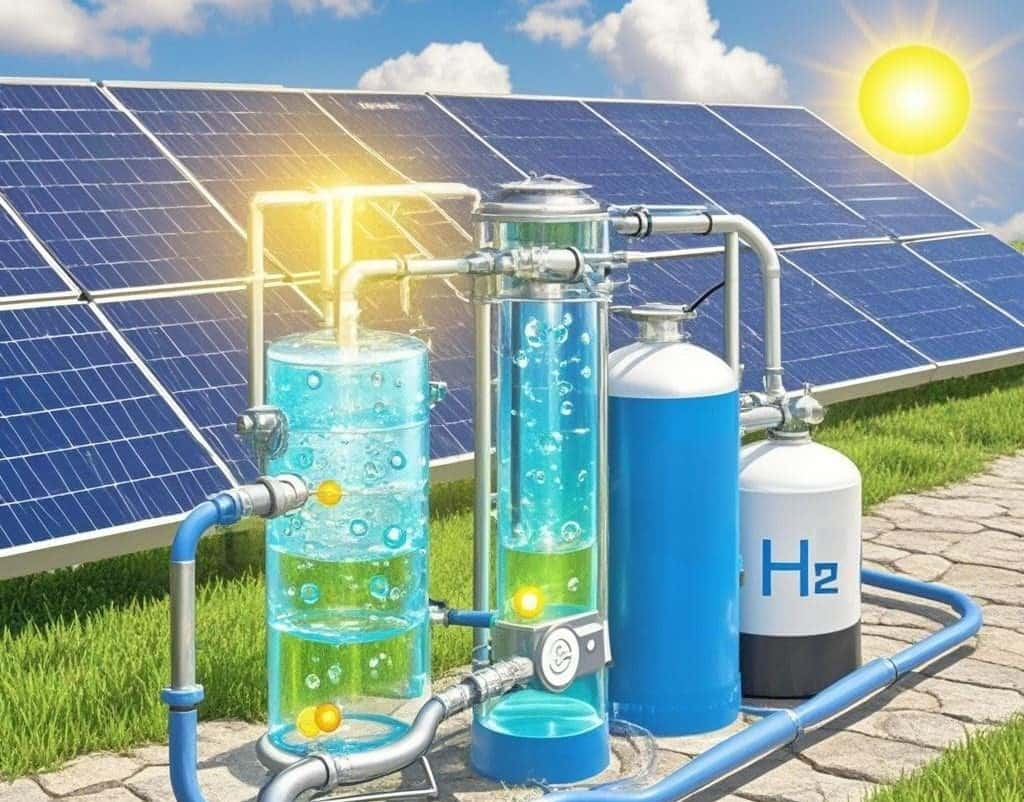
Solar Thermochemical Hydrogen Production
It offers the advantage of direct solar-to-hydrogen conversion. This eliminates the need for intermediate energy conversion steps in some cases, potentially increasing the overall energy efficiency of hydrogen generation. In solar thermochemical cycles, concentrated solar heat can be used to split water or other hydrogen-containing compounds. It has a very low environmental impact as it does not rely on fossil fuels and does not produce greenhouse gas emissions during the actual hydrogen production process.
However, the technology is still in the early stages of development and is relatively complex, requiring precise control of high-temperature solar concentrators and chemical reactors. There are significant technical challenges in maintaining stable operation under varying solar irradiation conditions. The capital cost for building solar thermochemical hydrogen production facilities is high due to the need for advanced materials, large-scale solar concentrators and specialised high-temperature reaction systems. Its market share is negligible, but it has significant potential for future growth. Scaling up solar thermochemical hydrogen production requires overcoming technical challenges, improving efficiency, and reducing costs through research and development.
Nuclear Hydrogen Production
Nuclear power can provide a large amount of heat in a continuous and reliable manner, which can be used for high-temperature steam electrolysis or thermochemical processes for hydrogen production. This can result in high production rates and stable hydrogen supply, independent of weather conditions like solar or wind-based methods. Nuclear hydrogen production has a relatively low carbon footprint compared to fossil fuel-based processes, contributing to the reduction of greenhouse gas emissions in the long term.
There are significant safety concerns associated with nuclear power plants, including the risk of nuclear accidents and the proper management of radioactive waste. These issues can lead to public opposition and regulatory hurdles in siting and operating nuclear-based hydrogen production facilities. The capital cost for building nuclear reactors for hydrogen production is extremely high, and the construction period is long, which can delay the implementation of such projects and increase financial risks.
The capital cost for electrolysis plants depends on the type of electrolyser technology used (e.g., alkaline, proton exchange membrane, or solid oxide). Generally, building large-scale electrolysis facilities can require significant investment. The availability and cost of renewable electricity on a large scale are key hurdles. There is also a need for further technological advancements to improve the efficiency and durability of electrolyser systems to make them more competitive with traditional hydrogen production methods.
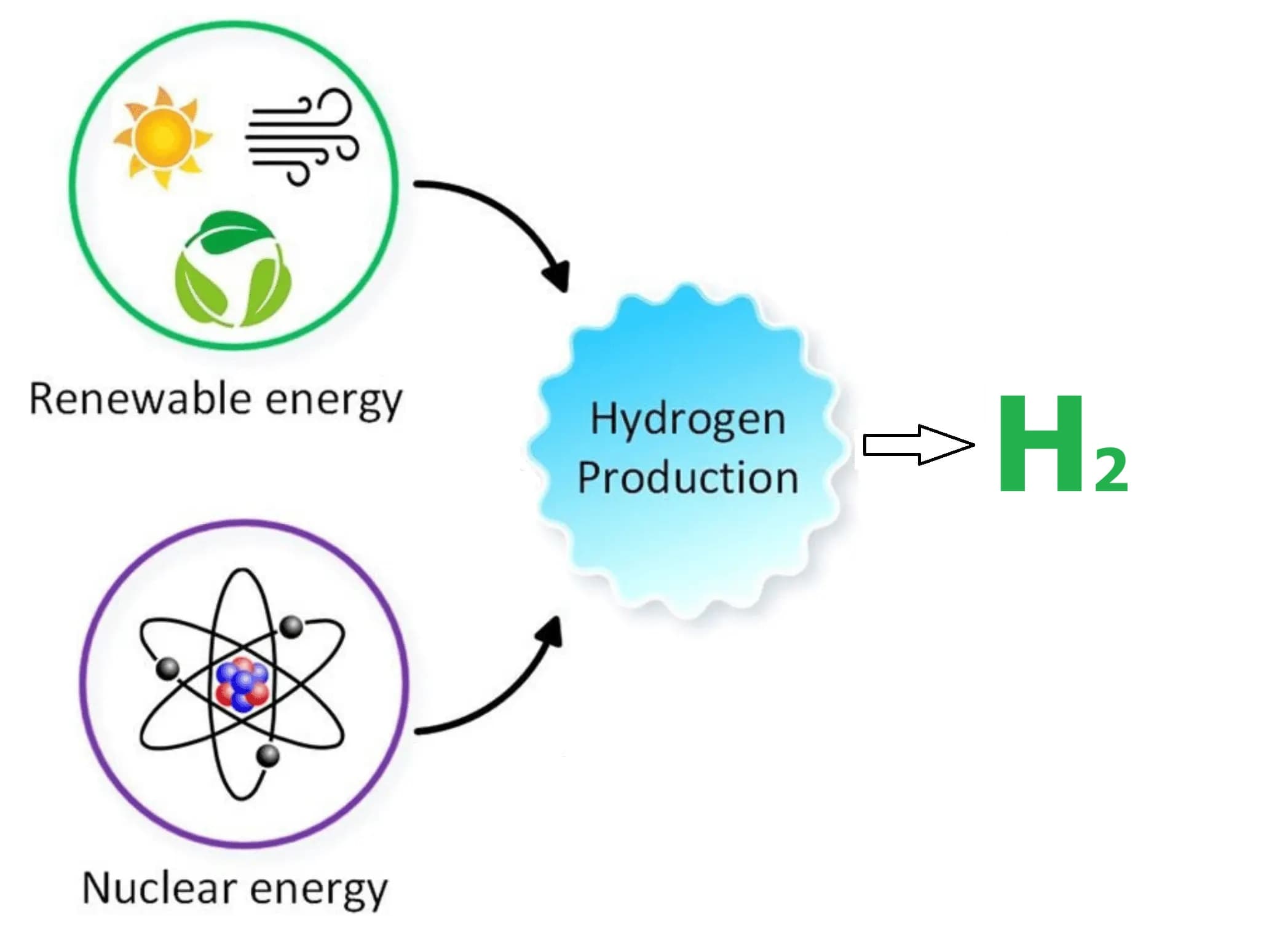
Hydrogen Production by Gasification
Coal Gasification is a process that converts coal into syngas, a mixture of hydrogen and carbon monoxide. This method is advantageous for regions with abundant coal reserves, offering a way to utilize this resource for hydrogen production. However, it is highly polluting, producing significant amounts of greenhouse gases and other pollutants.
Biomass Gasification is a renewable method that converts biomass into syngas, similar to coal gasification. Its primary advantage is the use of renewable feedstocks, reducing dependence on fossil fuels. However, it faces challenges related to feedstock availability and consistency, as well as the need for advanced gas cleaning technologies to remove impurities.
Building a gasification plant for ‘Brown’ hydrogen production involves high capital costs due to the need for specialised reactors, gas cleaning and separation units, and other associated infrastructure. The environmental regulations are becoming stricter worldwide, making it difficult to expand gasification based hydrogen production without significant investment in pollution control and carbon capture technologies. Moreover, the long-term viability of feedstock is being questioned. Additionally, as the demand for low-carbon hydrogen grows, there may be limitations in expanding gasification methods further, without addressing its environmental impact.
Conclusion
The current landscape of hydrogen generation technologies in the market is diverse, with each method having its own set of advantages and disadvantages, cost structures, maintenance requirements, market shares, and scaling-up challenges. While traditional fossil fuel-based methods like SMR and coal gasification dominate the market currently, the future is likely to see a shift towards renewable and low-carbon hydrogen production technologies such as electrolysis powered by renewable electricity, solar thermochemical, and potentially nuclear hydrogen production if the associated challenges can be overcome. The development and scaling-up of these cleaner hydrogen generation technologies will be crucial in realising the full potential of hydrogen as a key component in the global transition towards a sustainable energy future.
It is essential for policymakers, industry stakeholders, and researchers to work together to address the challenges faced by these technologies, whether it's through providing financial incentives for research and development, improving regulatory frameworks, or building the necessary infrastructure for the production, storage, and distribution of hydrogen. As the demand for clean hydrogen grows, a balanced approach that considers both the technical and economic aspects of these different hydrogen generation technologies will be necessary, to ensure a smooth and successful transition in the energy sector.
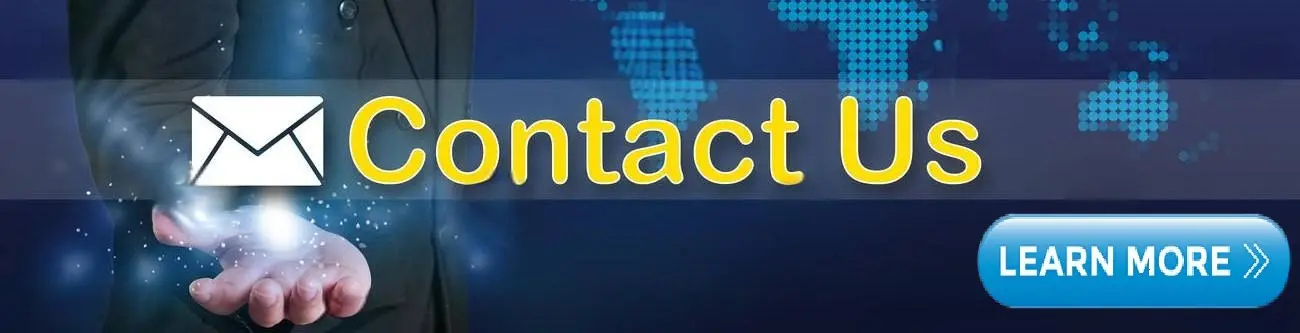
Back